Menu
Links
nils-uni@bluemer.name
|
|
Prof. Dr. Nils Blümer |
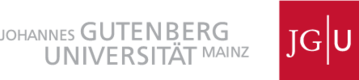
|
Attention: these pages are mostly outdated! For current information on Nils Blümer, see web pages at the KU.
Mott-Hubbard Metal-Insulator Transition and Optical Conductivity in High Dimensions
by Nils Blümer
(Shaker Verlag, ISBN 3-8322-2320-7)
Table of Contents
1.1.1 Solid State Theory for Crystals | | 6 |
1.1.2 Electronic Lattice Models | | 7 |
1.1.3 Wannier Representation | | 8 |
1.1.4 One-band Hubbard Model | | 9 |
1.2 Dynamical Mean-Field Theory | | 11 |
1.2.1 Limit Z→∞ for Spin Models | | 12 |
1.2.2 Limit Z→∞ for Fermions | | 13 |
1.2.3 Simplifications for the Hubbard Model in Z→∞ | | 15 |
1.3 Quantum Monte Carlo Algorithm | | 19 |
1.3.1 Wick's Theorem for the Discretized Impurity Problem | | 19 |
1.3.2 Monte Carlo Importance Sampling | | 21 |
1.4 Maximum Entropy Method | | 23 |
2 Lattice and Density of States | | 27 |
2.1 Hypercubic Lattice and Extensions | | 29 |
2.1.1 Definitions and Analytical Considerations | | 29 |
2.1.2 Numerical Results | | 32 |
2.1.3 Magnetic Frustration and Asymmetry of the DOS | | 38 |
2.2 Bethe Lattice, RPE, and Disorder | | 39 |
2.2.1 Bethe Tree, Cayley Tree, and Husimi Cactus | | 40 |
2.2.2 Renormalized Perturbation Expansion | | 43 |
2.3 General Density of States in d=∞ | | 47 |
2.4 Redefinition of the Bethe Lattice | | 53 |
2.4.2 Truncating the Hopping Range | | 57 |
2.4.3 Finite Dimensionality | | 59 |
2.4.4 Application to Asymmetric Model DOS | | 62 |
3 Mott Metal-Insulator Transition in the d→∞ Hubbard Model | | 67 |
3.2 Characterization of Phase Transitions within the DMFT | | 73 |
3.2.1 Transitions of First or Higher Order | | 73 |
3.2.2 Convergence of Fixed Point Methods | | 75 |
3.3 Phase Diagram: Development until 1999 | | 79 |
3.4 Discussion of QMC Algorithms | | 82 |
3.4.1 Fourier Transformation and Smoothing | | 84 |
3.4.2 Overrelaxation and Sweeping Strategies | | 89 |
3.4.3 Estimation of Errors | | 90 |
3.5 Results: Coexistence Region | | 93 |
3.5.1 Choice of Observables and Extrapolation | | 93 |
3.5.2 Properties of the Insulating Phase | | 96 |
3.5.3 Internal Energy | | 100 |
3.5.4 Coexistence Phase Diagram | | 108 |
3.5.5 Double Occupancy | | 113 |
3.6 Results: Thermodynamic Phase Transition Line | | 121 |
3.6.1 Differential Equation for dUc/dT and Linearization | | 121 |
3.6.2 Low-temperature Asymptotics of U(T) | | 125 |
3.6.3 Full Phase Diagram | | 131 |
3.6.4 Implications of Partial Frustration | | 138 |
3.7 Landau Theory and Criticality | | 142 |
3.7.1 Free Energy Functional for the Bethe Lattice | | 143 |
3.7.2 Direct Evaluation of Free Energy Differences | | 144 |
3.7.3 Critical Behavior Near the MIT | | 146 |
3.8.1 Maximum Entropy Method for Spectral Functions | | 155 |
3.8.2 Algorithmic Choices and Numerical Tests | | 160 |
3.8.3 Numerical Results for the Bethe DOS | | 166 |
4 Optical Conductivity | | 175 |
4.1 Definition and General Properties of the Optical Conductivity | | 176 |
4.1.1 Connection between Conductivity and Reflectivity | | 177 |
4.1.2 Optical f-sum Rules | | 179 |
4.1.4 Impact of Electronic Model Abstractions | | 183 |
4.2.1 Kubo Formalism in the Continuum | | 186 |
4.2.2 Kubo Formalism on a Lattice | | 188 |
4.2.3 General Confirmation of the f-sum Rule | | 190 |
4.3 Optical Conductivity in the Limit d→∞ | | 192 |
4.3.1 Optical Conductivity for the Hypercubic Lattice | | 194 |
4.3.2 f-sum Rule within the DMFT | | 195 |
4.3.3 f-sum Rule and General Dispersion Formalism | | 197 |
4.4 Optical Conductivity for the Bethe Lattice | | 198 |
4.4.1 Treelike Layout of the Bethe Lattice | | 200 |
4.4.2 Single-Chain Stacked Bethe Lattice | | 204 |
4.4.3 Periodically Stacked Lattices | | 209 |
4.4.4 Offdiagonal Disorder | | 211 |
4.4.5 General Dispersion Method | | 213 |
4.5.1 Coherent versus Incoherent Transport in High Dimensions | | 216 |
4.5.2 Optical Conductivity in Finite Dimensions | | 219 |
4.5.3 Impact of Frustration by t-t' Hopping | | 222 |
4.6 QMC Results for the Bethe Lattice | | 225 |
4.6.1 Numerical Procedure for QMC Data | | 226 |
4.6.2 Results: Self-Energy on the Real Axis | | 230 |
4.6.3 Results: Optical Conductivity | | 234 |
5 Realistic Modeling of Strongly Correlated Materials | | 245 |
5.4 Results for La1-xSrxTiO3 | | 255 |
5.4.1 Density of States and Photoemission Spectra | | 255 |
5.4.2 Influence of Discretization Errors | | 261 |
5.4.3 Optical Conductivity | | 268 |
A Additions to ``Models and Methods'' | | 281 |
A.1 Extensions of the Hubbard Model | | 281 |
A.2 Characterization of Generic Momenta | | 284 |
A.3 DCA, CDMFT, and RDA | | 286 |
B Hyperdiamond Lattice | | 291 |
C Fourier-Transforming Imaginary-Time Green Functions | | 297 |
D Linear Response to Electromagnetic Fields | | 305 |
D.1 Electromagnetic Interaction Hamiltonian and Choice of Gauge | | 305 |
D.2 Linear Response Theory | | 306 |
| |
|